A Brief History of Accelerators
A brief history of the development of particle accelerators and the key discoveries they enabled.
BLOG
8/19/20244 min read
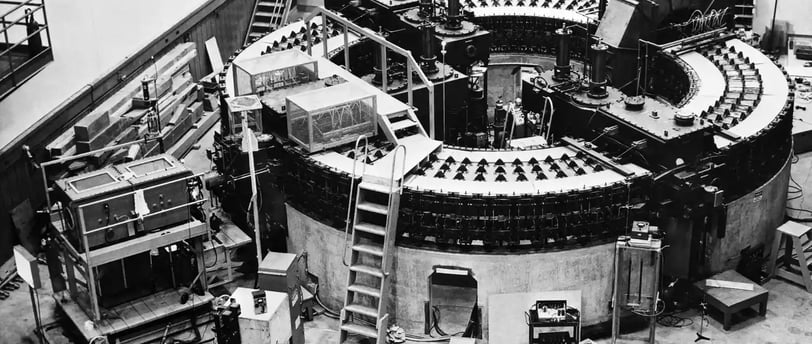
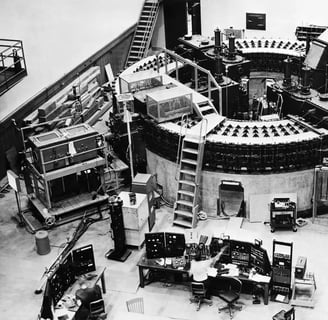
Today, particle accelerators hum across the globe, propelling beams of charged particles that serve a plethora of incredible purposes. At the largest scale, multibillion-dollar accelerator facilities push the boundaries of particle and nuclear physics, probing interactions hidden deep within the fabric of our universe. These accelerators also produce coherent sources of synchrotron radiation, enabling groundbreaking research in physics, chemistry, biology, and materials science. Every day, they are vital in medical facilities worldwide—sterilizing medical equipment, producing radioisotopes, and destroying cancerous tumors. Moreover, accelerators in the form of ion implanters are critical in fabricating the semiconductors that power our computers and electronics. These examples represent just a few of the many crucial applications accelerators have in our modern world. Tomorrow, we can anticipate even more advanced versions of these delightful machines, making accelerators even more prevalent and integrated into our daily lives.
In this mini blog post, I just want to sketch a brief history of how we got here and of a few key advancements and discoveries made in the exciting world of accelerator physics. What follows might serve as an appetizer on the subject, with supplemental reading and exploration links at the end.
Humanity has observed the effects of moving charged particles long before understanding the structure and nature of the particles themselves. The Aurora Borealis, lightning strikes, and various chemical reactions are all the results of charged particles changing their trajectories—sometimes with dramatic consequences. In the early to mid-1800s, figures like Oersted, Ampere, Faraday, and Maxwell worked to formalize our understanding of electromagnetism, laying the foundational physics necessary to manipulate these charged particles. The first true particle accelerator soon followed in the form of a cathode ray tube. This early design accelerated electrons by creating an electrostatic potential difference between the two ends of an evacuated tube. Physics undergraduates around the world study the landmark discoveries made possible by this innovation, including J.J. Thomson’s realization that the atom contained a constituent just 1/1,000th its size—the electron
The early 20th century witnessed the birth of nuclear physics, marked by the advent of belt-charged electrostatic generators. Pioneering devices like the Van de Graaff accelerator and the Cockcroft-Walton generator enabled particles to be accelerated to relativistic speeds. These accelerators further probed the atom’s structure, laying the groundwork for the quantum theory that would revolutionize physics. In 1931, Lawrence and Livingston constructed the first circular accelerator—a 9-inch cyclotron—which sparked a wave of new circular accelerator designs. Among these was the betatron, operational by 1940, which famously demonstrated fast transverse oscillations in weak focusing, known as betatron oscillations—a key principle in beam dynamics.
The practical applications of early accelerator technology were soon showcased by the manufacture of the first CRT televisions in the 1930s and 1940s. The cathode ray tube, initially developed for scientific experiments, became a key component in consumer electronics, leading to the widespread use of televisions. World War II further galvanized innovation in this field. The Alvarez drift tube linear accelerator leveraged developments in radar technology to exploit high-power microwave sources, marking the birth of RF linacs. By circumventing electrostatic breakdown, RF linacs enabled us to reach even higher energies, remaining a cornerstone of accelerator physics. Post-war advancements saw the cyclotron evolve into the synchrotron, which combined the principles of linear and circular accelerators, utilizing radio-frequency acceleration while maintaining particles on a fixed-radius orbit.
In the decades that followed, synchrotrons came to dominate high-energy regimes, steadily increasing in power. This highly successful design has been scaled up over the years, culminating in the current state-of-the-art Large Hadron Collider, which reaches energies on the order of 10 TeV. The synchrotron not only advances particle physics but also produces synchrotron radiation, a type of braking radiation critical for high-energy research and the foundation for free-electron lasers. While synchrotron radiation limits electron synchrotron energy, it is invaluable for modern science's high-quality, high-energy applications.
Now in the modern day, we continue the historical trend of pushing to construct accelerators capable of achieving higher and higher energies. At the same time, we are also pursuing novel approaches to miniaturize the technology, reduce costs, and discover more specialized applications. Emerging technologies, such as laser-driven, plasma-based, and ultra-high-intensity beams, hold the promise of meeting these challenges, potentially revolutionizing the field once again.
As we look to the future, the enduring influence of accelerators on scientific and technological progress is undeniable. They will continue to play a critical role in high-energy physics, allowing us to probe the deepest mysteries of the universe. Beyond fundamental research, accelerators are indispensable in diverse fields: from the synchrotron radiation sources crucial to materials science and biology, to ion implantation techniques essential for semiconductor manufacturing. Their applications extend into industrial processes, food sterilization, waste management, and advanced medical treatments like radiotherapy.
With their diverse application portfolio and track record of discovery, accelerators have joined the ranks of enduring and accomplished instruments like the telescope and microscope, making profound contributions to scientific understanding and modern life. As with these devices, the state of the art in accelerators continues to advance, pushing the envelope of discovery, guiding technological evolution as well as particle beams, and accelerating us into our future.
References
Rosenzweig, J. B. (2003). Fundamentals of Beam Physics. Oxford University Press.
Safarik, K. (Ed.). (2003). The Early History of Particle Accelerators. In The CAS Particle Accelerators School, Zakopane. Retrieved from https://cas.web.cern.ch/sites/default/files/lectures/zakopane-2006/tazzari-history.pdf
Bryant, P. J. (2000). A Brief History and Review of Accelerators. CERN, Geneva, Switzerland. Retrieved from https://cds.cern.ch/record/261062/files/p1_2.pdf
Wilson, E. (2001). An Introduction to Particle Accelerators. Oxford University Press. Retrieved from https://academic.oup.com/book/11694/chapter/160639804